Abstract
Stochastic spatial predator-prey competition models represent paradigmatic systems to understand the emergence of biodiversity and the stability of ecosystems. We aim to clarify the relationship and connections between interacting three-species models and the classic two-species Lotka-Volterra (LV) model that entails predator-prey coexistence with long-lived population oscillations. To this end, we utilize mean-field theory and Monte Carlo simulations on two-dimensional square lattices to explore the temporal evolution characteristics of two different interacting three-species predator-prey systems, namely: (1) a cyclic rock-paper-scissors (RPS) model with conserved total particle number but strongly asymmetric reaction rates that lets the system evolve towards one “corner” of configuration space; (2) a hierarchical “food chain” where an additional intermediate species is inserted between the predator and prey in the LV model. For the asymmetric cyclic model variant (1), we demonstrate that the evolutionary properties of both minority species in the (quasi-)steady state of this stochastic spatial three-species “corner” RPS model are well approximated by the two-species LV system, with its emerging characteristic features of localized population clustering, persistent oscillatory dynamics, correlated spatio-temporal patterns, and fitness enhancement through quenched spatial disorder in the predation rates. In contrast, we could not identify any regime where the hierarchical three-species model (2) would reduce to the two-species LV system. In the presence of pair exchange processes, the system remains essentially well-mixed, and we generally find the Monte Carlo simulation results for the spatially extended hierarchical model (2) to be consistent with the predictions from the corresponding mean-field rate equations. If spreading occurs only through nearest-neighbor hopping, small population clusters emerge; yet the requirement of an intermediate species cluster obviously disrupts spatio-temporal correlations between predator and prey, and correspondingly eliminates many of the intriguing fluctuation phenomena that characterize the stochastic spatial LV system.
Similar content being viewed by others
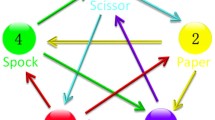
References
J.M. Smith, Evolution and the Theory of Games (Cambridge University Press, Cambridge, UK, 1982)
J. Hofbauer, K. Sigmund, Evolutionary Games and Population Dynamics (Cambridge University Press, Cambridge, UK, 1998)
M.A. Nowak, Evolutionary Dynamics (Belknap Press, Cambridge, USA, 2006)
R.M. May, W.J. Leonard, SIAM J. Appl. Math. 29, 243 (1975)
R.M. May, Stability and Complexity in Model Ecosystems (Cambridge University Press, Cambridge, UK, 1974)
J.M. Smith, Models in Ecology (Cambridge University Press, Cambridge, UK, 1974)
R.E. Michod, Darwinian Dynamics (Princeton University Press, Princeton, USA, 2000)
R.V. Sole, J. Basecompte, Self-Organization in Complex Ecosystems (Princeton University Press, Princeton, USA, 2006)
D. Neal, Introduction to Population Biology (Cambridge University Press, Cambridge, UK, 2004)
A.J. Lotka, Proc. Natl. Acad. Sci. USA 6, 410 (1920)
A.J. Lotka, J. Amer. Chem. Soc. 42, 1595 (1920)
V. Volterra, Mem. Accad. Lincei 2, 31 (1926)
V. Volterra, Lecons sur la théorie mathématique de la lutte pour la vie (Gauthiers-Villars, Paris, 1931)
R. Monetti, A.F. Rozenfeld, E.V. Albano, Physica A 283, 52 (2000)
E. Bettelheim, O.A. Nadav, N.M. Shnerb, Physica E 9, 600 (2001)
M. Droz, A. Pekalski, Phys. Rev. E 63, 051909 (2001)
T. Antal, M. Droz, Phys. Rev. E 63, 056119 (2001)
M. Kowalik, A. Lipowski, A.L. Ferreira, Phys. Rev. E 66, 066107 (2002)
A.J. McKane, T.J. Newman, Phys. Rev. Lett. 94, 218102 (2005)
M.J. Washenberger, M. Mobilia, U.C. Täuber, J. Phys.: Condens. Matter 19, 065139 (2007)
M. Mobilia, I.T. Georgiev, U.C. Täuber, J. Stat. Phys. 128, 447 (2007)
G. Szabó, G. Fáth, Phys. Rep. 446, 97 (2007)
S. Venkat, M. Pleimling, Phys. Rev. E 81, 021917 (2010)
L. Frachebourg, P.L. Krapivsky, E. Ben-Naim, Phys. Rev. E 54, 6186 (1996)
B. Sinervo, C.M. Lively, Nature 380, 240 (1996)
K.R. Zamudio, B. Sinervo, Proc. Natl. Acad. Sci. USA 97, 14427 (2000)
B. Kerr, M.A. Riley, M.W. Feldman, B.J.M. Bohannan, Nature 418, 171 (2002)
T. Reichenbach, M. Mobilia, E. Frey, Nature 448, 1046 (2007)
T. Reichenbach, M. Mobilia, E. Frey, Phys. Rev. Lett. 99, 238105 (2007)
T. Reichenbach, M. Mobilia, E. Frey, J. Theor. Biol. 254, 368 (2008)
J.D. Murray, Mathematical Biology (Springer, New York, USA, 2002), Vols. I, II
U. Dobramysl, U.C. Täuber, Phys. Rev. Lett. 101, 258102 (2008)
A. Lipowski, D. Lipowska, Physica A 276, 456 (2000)
A.F. Rozenfeld, E.V. Albano, Physica A 266, 322 (1999)
J.E. Satulovsky, T. Tomé, Phys. Rev. E 49, 5073 (1994)
N. Boccara, O. Roblin, M. Roger, Phys. Rev. E 50, 4531 (1994)
A. Lipowski, Phys. Rev. E 60, 5179 (1999)
D. Panja, Phys. Rep. 393, 87 (2004)
L. O’Malley, B. Kozma, G. Korniss, Z. Rácz, T. Caraco, Phys. Rev. E 74, 041116 (2006)
S.R. Dunbar, J. Math. Biol. 17, 11 (1983)
J. Sherratt, B.T. Eagen, M.A. Lewis, Philos. Trans. R. Soc. Lond. B 352, 21 (1997)
M.A.M. de Aguiar, E.M. Rauch, Y. Bar-Yam, J. Stat. Phys. 114, 1417 (2004)
A. Provata, G. Nicolis, F. Baras, J. Chem. Phys. 110, 8361 (1999)
M. Mobilia, I.T. Georgiev, U.C. Täuber, Phys. Rev. E 73, 040903(R) (2006)
T. Reichenbach, M. Mobilia, E. Frey, Banach Center Publications 80, 259 (2008)
T. Reichenbach, M. Mobilia, E. Frey, Phys. Rev. E 74, 051907 (2006)
M. Berr, T. Reichenbach, M. Schottenloer, E. Frey, Phys. Rev. Lett. 102, 048102 (2009)
K.I. Tainaka, Phys. Rev. E 50, 3401 (1994)
G. Szabó, A. Szolnoki, Phys. Rev. E 65, 036115 (2002)
M. Perc, A. Szolnoki, G. Szabó, Phys. Rev. E 75, 052102 (2007)
G.A. Tsekouras, A. Provata, Phys. Rev. E 65, 016204 (2001)
Q. He, M. Mobilia, U.C. Täuber, Eur. Phys. J. B 82, 97 (2011)
M. Frean, E.R. Abraham, Proc. R. Soc. Lond. B 268, 1323 (2001)
Q. He, M. Mobilia, U.C. Täuber, Phys. Rev. E 82, 051909 (2010)
M. Peltomäki, M. Alava, Phys. Rev. E 78, 031906 (2008)
R.K.P. Zia, e-print arXiv:1101.0018 (2011)
U.C. Täuber, J. Phys. Conf. Ser. 319, 012019 (2011)
C.H. Durney, S.O. Case, M. Pleimling, R.K.P. Zia, Phys. Rev. E 83, 051108 (2011)
A. Dobrinevski, E. Frey, e-print arXiv:1001.5235 (2010)
Author information
Authors and Affiliations
Corresponding author
Rights and permissions
About this article
Cite this article
He, Q., Täuber, U.C. & Zia, R.K.P. On the relationship between cyclic and hierarchical three-species predator-prey systems and the two-species Lotka-Volterra model. Eur. Phys. J. B 85, 141 (2012). https://doi.org/10.1140/epjb/e2012-20918-4
Received:
Published:
DOI: https://doi.org/10.1140/epjb/e2012-20918-4